Even though we can’t see them, we live our lives surrounded by neutrinos.
These tiny, high-energy cosmic particles are often referred to as “ghostly” because they are extremely volatile, or vaporous, particles that can pass through any kind of matter without changing.
Neutrinos have almost no mass and can travel through the most extreme environments, like stars, planets and entire galaxies, and not change their structure at all.
“Neutrinos are these crazy, weird particles. No one ordered all of the crazy features they have, they just showed up, and we’re still trying to figure out what they are,” said Peter Gorham, professor of physics at the University of Hawai’i at Mānoa. “The universe is chock full of them. A billion of them go through our noses every second and we don’t even feel it.”
Data collected bydetectors in Antarctica and teams ofdevotedscientists have suggested new insights about neutrinos, as well as some anomalies that can’t be explained.
The data have not, however, proved the existence of a parallel universe, said Gorham, who is also the principal investigator of ANITA, short for Antarctic Impulsive Transient Antenna,a high energy particle detector in Antarctica.
Why the confusion?
Recent articles by other media outlets about some of the unusual data picked up by ANITA have conflated theories by physicists outside the experiment, with that of researchers using the instrument. One of those theories involves the idea of a parallel universe. Gorham noted, however, that the team of researchers directly involved did not posit this theory.
What’s exciting about the quest to understand neutrinos, strange particles detected on Earth that are prevalent in the universe, is that it’s ongoing and evolving — and has only just begun, really. While it may take decades to truly understand what they can tell us, scientists believe that tracing neutrinos back to their sources can shed light on their origins in the distant universe.
Some physicists say this is leading to a new era of neutrino astronomy. Strange as they are, neutrinos can help us understand the universe in new ways. Here’s why.
Neutrinos on Earth
Detecting neutrinos on Earth can allow them to be traced back to their sources, which could reveal more about the origin of mysterious cosmic rays.
Cosmic rays, the most highly energetic particles in the universe, bombard Earth from space. Their ionizing particles in our atmosphere were detected for the first time more than 100 years ago, in 1912, by physicist Victor Hess. He determined that they came from space.
Cosmic rays are mostly made up of protons or atomic nuclei, and they’re launched across the universe because whatever produces them is such a powerful particle accelerator that it dwarfs the capabilities of the Large Hadron Collider near Geneva.
But these rays have puzzled scientists ever since their discovery. Where do they come from, and what creates and launches them? Neutrinos could tell us.
“To me, neutrinos are the key to learning about the universe at its most extreme,” said Abigail Vieregg, an associate professor in the department of physics at the University of Chicago, Enrico Fermi Institute and Kavli Institute for Cosmological Physics.
“They will let us learn about the sources in the universe that make the most energetic stuff that are raining down on us, like cosmic rays. They travel really far through the universe. While other particles get mixed up in the dust of universe, neutrinos are a pure, beautiful signal that point to where they came from. I think about them as a perfect messenger, and they tell us about sources from far away.”
But Vieregg said that the beauty of the pure signal provided by neutrinos serves as a double-edged sword: They’re difficult to detect because they don’t often interact with their surroundings.
Neutrinos do interact with ice, however. And the highest concentration of ice on our planet can be found in Antarctica.
Neutrino research has been occurring for some time with exciting results in recent years. In 2018, scientists were able to trace the origins of a neutrino that traveled 3.7 billion light-years to Earth with the help of sensors deep in the Antarctic ice in the IceCube detector at the South Pole Neutrino Observatory.
The IceCube detector became operational in 2010. Largely funded by the National Science Foundation, IceCube was built to detect high-energy neutrinos. It’s the largest detector of its kind.
To build it, workers drilled 86 holes in the ice, each 1.5 miles deep, and spread a network of 5,160 light sensors over a grid of 1 cubic kilometer. It’s operated by a team based at the University of Wisconsin-Madison, but the IceCube collaboration itself includes 300 scientists and 49 institutions.
In 2013, IceCube discovered the first neutrinos with higher energy from beyond our galaxy and continued to observe a number of high-energy neutrinos without tracing them until the summer of 2018. IceCube monitors the sky and detects about 200 neutrinos per day, but most are low energy, created when cosmic rays interact with Earth’s atmosphere.
But it’s not the only detector trying to understand neutrinos among the ice of Antarctica.
The ANITA experiments
While IceCube’s sensors are deep beneath the ice, ANITA flies high above it.
ANITA’s roots go back 20 years, principal investigator Gorham said. He and physicist David Saltzberg, professor and chair of the physics and astronomy department at the University of California, Los Angeles, worked on research and experiments to confirm a prediction by Russian-Armenian physicist Gurgen Askaryan. He suggested that when a high energy particle interacts in a solid medium like ice, it creates a secondary shower of particles known as an electromagnetic cascade.
This effect could create almost a mini-lightning bolt inside the material in which it interacts. For example, if this particle managed to penetrate and interact deep within Antarctic ice, a visible blue flash would be created by a lightning bolt between 20 to 30 feet long. That blue light is known as Cherenkov radiation.
Askaryan realized that in addition to the blue light, a burst of radio emissions would also take place. In 2001, Gorham and Saltzberg succeeded in measuring this secondary burst of radio waves in a lab experiment for the first time, proving Askaryan’s 1962 prediction was correct.
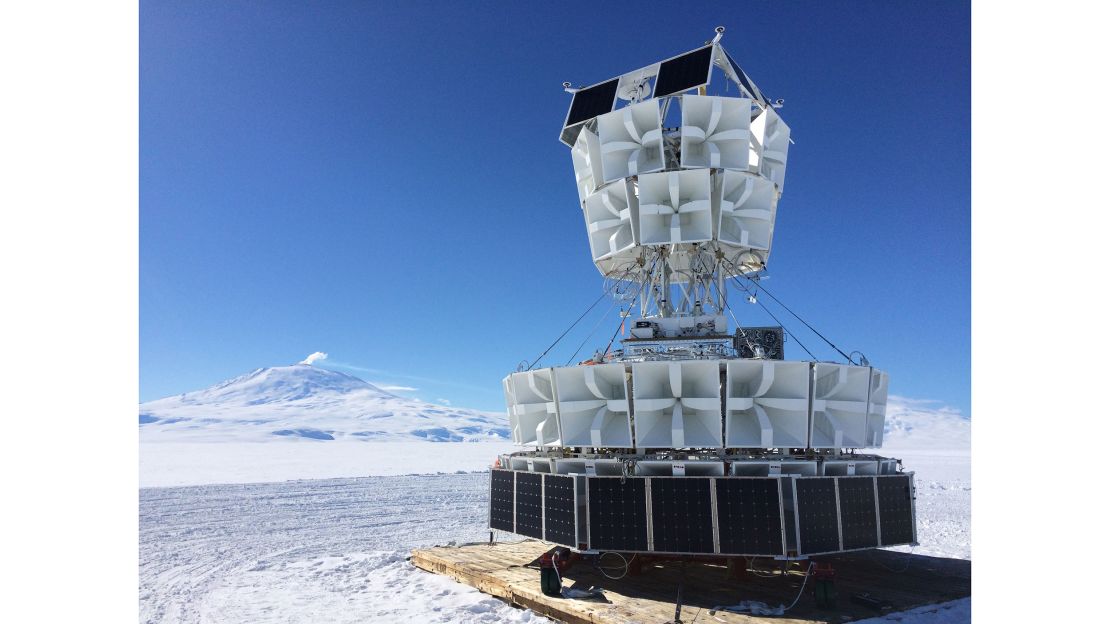
They were attracted to the idea of using ice to detect the interaction of ultra-high energy cosmic-ray neutrinos. Radio waves also spread farther in cold ice than optical light. It’s how geophysicists and glaciologists can probe the underside of glaciers and use radar to look through thick ice sheets.
ANITA became the logical next step, Gorham said.
“If we want to see neutrinos, maybe we should fly a radio detector above Antarctica and wait for those radio bursts to appear,” Gorham told CNN, recalling a conversation he had with Saltzberg about designing ANITA.
They designed the ANITA instrument as a radio telescope to detect high-energy neutrinos that could be attached to a scientific balloon flying over Antarctica. Each flight lasted about a month, with the first occurring in 2006 and the last flight occurring in late 2016. ANITA was funded by NASA.
ANITA’s anomalies
So far, ANITA’s investigators have seen no convincing evidence of neutrinos — in the traditional definition that they were looking for — via cascade bursts in the ice sheets, Gorham said.
But it has detected something intriguing. ANITA was designed to detect cosmic ray particle showers through radio signals either on their way down to the ice, or traveling back up after bouncing off of it.
And during its flights, ANITA found high-energy fountains of particles coming from the ice, a kind of upside-down shower of cosmic rays.
Neutrinos come in three flavors, essentially: electron neutrinos, muon neutrinos and tau neutrinos. Although these are charged particle cousins with unique properties, there are limited ways of distinguishing between them, Gorham said.
The tau neutrino has an interesting feature in that it regenerates. These neutrinos have short lifetimes, but when they decay at high energies, they produce another tau neutrino, as well as a particle called a tau lepton, and so forth. This means they are more likely to get through than other neutrinos.
And if this decay occurs in the atmosphere, it creates a cascade of particles like a cosmic ray.
Coincidentally, ANITA is sensitive to ultra-high energy cosmic rays that rain down on Earth and create a radio burst that acts like a flashlight beam of radio waves.
ANITA’s unique perspective means that it’s looking down at the ice, in the same direction of a beam that would rain down and reflect off the surface of the ice.
When ANITA watches a cosmic ray, the flashlight beam is really a burst of radio waves a billionth of a second long that can be mapped, like a wave, with a certain order of crests and troughs to show how it reflects off the ice — likely in reverse.
But twice in their data from ANITA flights, the researchers spotted what looked to be the eruption of a high energy particle coming up through the ice. The crest and trough order were not reversed.
Low energy neutrinos may be able to pass through our planet unscathed, but high energy neutrinos would experience more of a hard stop — and not erupt from the ice.
The researchers had a thought. Perhaps if a tau neutrino was spreading through the Earth and escaping the ice, regenerating along the way, it would create a similar radio flashlight beam pointing up at ANITA.
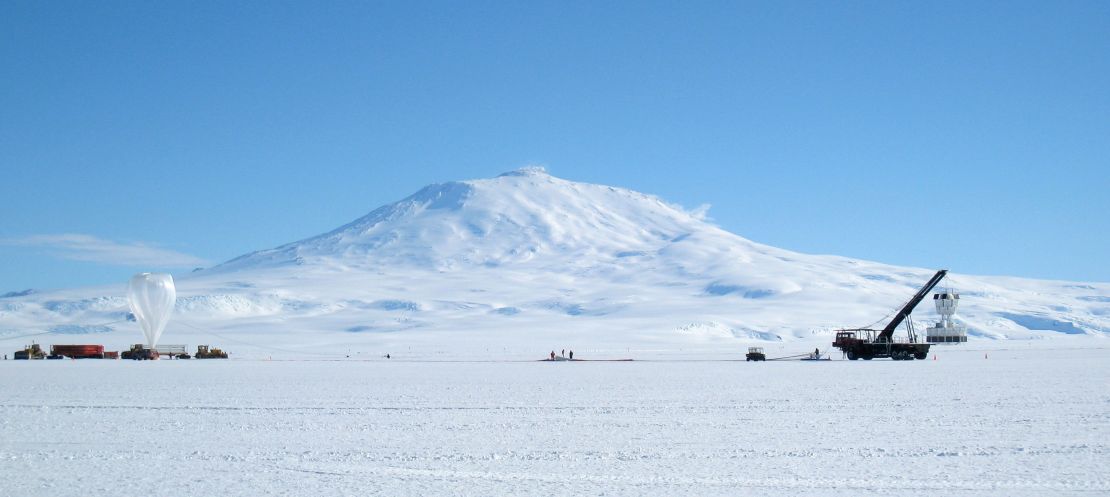
But could a tau neutrino, even with its regenerations, go this deep through the Earth and produce a shower up through the ice pointing at ANITA? Gorham and his colleagues didn’t believe so — the neutrino would run out of gas, so to speak.
It was just the beginning of a mystery, with a signature of something too deep to be a neutrino in the normal sense, and with no explanation beyond that.
ANITA and IceCube
Gorham and his colleagues are still going through data from the fourth flight of ANITA. They have used a blind analysis, where they blind themselves to certain features in the data, so they can’t simply produce the things they want to see.
Up through the third flight, they have two events that may signal an unusual particle, or one thatmay be explainable through more mundane physics that they don’t understand, Gorham said.
ANITA and IceCube actually complement each other because they can see and detect things differently.
ANITA’s 48 antennas — installed on a 25 foot tall balloon gondola –point toward the ice from above in a search for radio waves created by high-energy neutrinos when they crash into an atom in the ice.
When a neutrino interacts with the nucleus of an atom, it creates a secondary charged particle, producing a cone of blue light that can be detected and mapped by IceCube’s light sensor grid and traced back to its source.
IceCube has followed up on the detections made by ANITA to determine if it could see them.
“IceCube does not see these events despite the fact that our sensitivity is more than one order of magnitude higher than ANITA,” said Francis Halzen, IceCube principal investigator and Hilldale and Gregory Breit professor at the University of Wisconsin-Madison, in an email to CNN.
“We published our conclusions, and the ANITA collaboration does not challenge them. It is left as an unresolved problem.
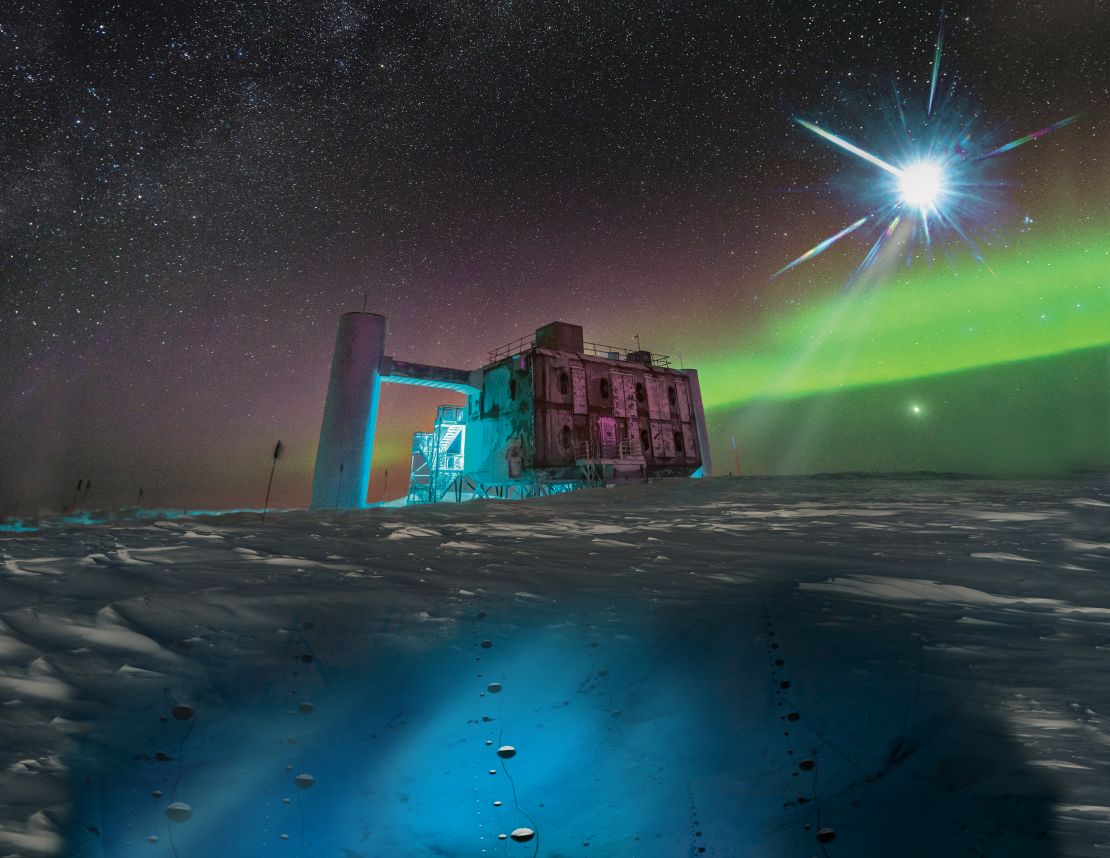
“It is clear however that these events cannot be neutrinos that have traversed the Earth and somehow escaped detection by IceCube,” Halzen said.
“The conclusion is that they must have come from above and have reflected off the ice into the ANITA detector without leaving any signature in the deep ice where IceCube would have recorded them.
“ANITA observes such events routinely; they are cosmic rays reflecting off the ice surface. The anomaly is that two events did not change polarization when reflected. This is either the result of new physics, or of some unanticipated way that particles can reflect from the polar ice cap’s surface.”
Gorham said that there is inevitable tension between the results of ANITA and IceCube because they aren’t seeing the same things and they don’t know how to resolve that yet. They are very different detectors using different methods.
“In physics, that’s a good thing,” Gorham said. “It’s great to approach a problem from different angles using different techniques. Sometimes, it’s the way you get the best view of something.”
Creating confusion about a parallel universe
In April, New Scientist published an article about neutrino research and the anomalous detections by ANITA, while also including theories and suggestions of other well-respected physicists not associated with Gorham’s research.
This included a 2018 study by physicist Luis A. Anchordoqui, a professor in the department of physics and astronomy at Lehman College, which applied physicist Neil Turok’s concept of a parallel universe, called a CPT symmetric universe, as a possible physics explanation for the anomalous events of the two high energy particle detections witnessed by ANITA, Gorham said. Turok is the director emeritus and holds the Mike and Ophelia Lazaridis Niels Bohr chair in Theoretical Physics at Perimeter Institute for Theoretical Physics.
There is much more detail to Turok and Anchordoqui’s theories, in both the article and their studies.
When other mediaoutlets picked up the story, what followed was a conflation of theories like the parallel universe idea being directly associated with Gorham’s research and statements regarding ANITA.
“NASA and Gorham’s team do not believe the gathered data is proof of a parallel universe,” according to a statement from the agency. “NASA relies on the scientific community peer-review process through research journals and publications. Tabloids have misleadingly connected NASA and Gorham’s experimental work, which identified some anomalies in the data, to a theory proposed by outside physicists not connected to the work. Gorham believes there are more plausible, easier explanations to the anomalies.”
The University of Hawaiʻi at Mānoa has also put out a release addressing the issue.
Gorham said from his own gut level at this point in their work, none of the possible explanations of the anomalies are completely satisfying. He cited the Occam’s razor approach: “The most likely explanation of any phenomenon is the simplest one,” Gorham said. “Right now, we don’t have a simple one.”
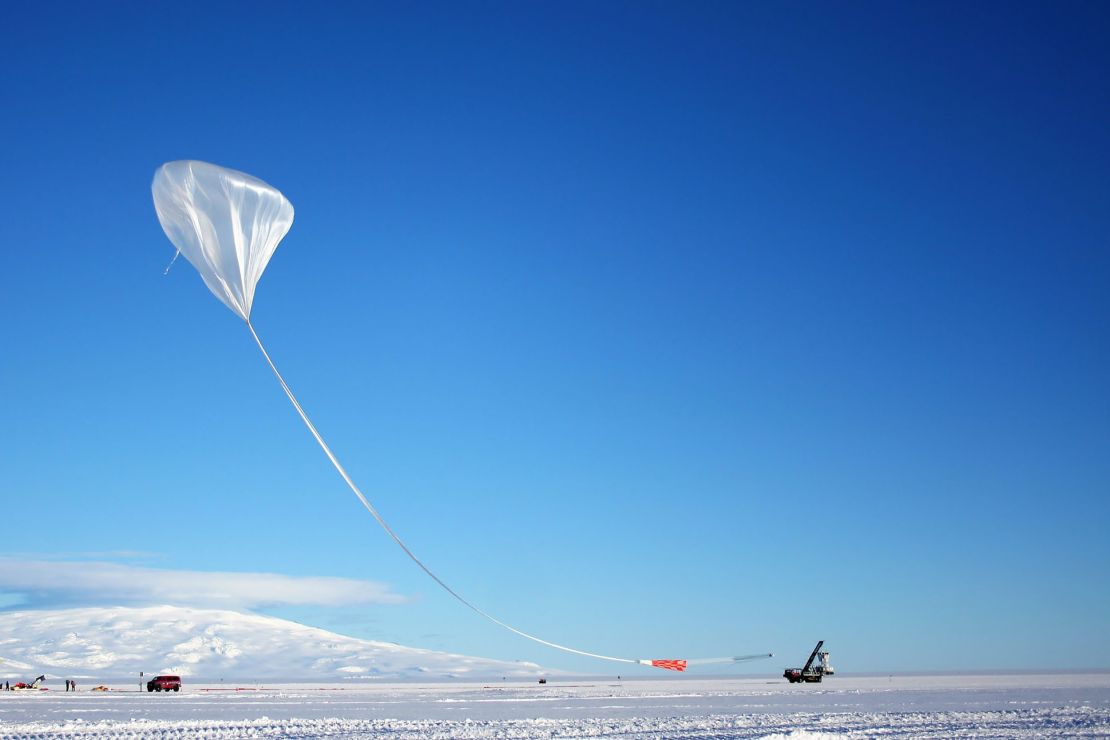
Exotic physics, like the parallel universe idea, don’t provide simple explanations either.
“I have no problem with that kind of free speculation,” Gorham said. “I think it’s wonderful for physicists to explore all the possibilities in any anomalous result. It’s still a valid explanation, but in my estimation, it’s probably not the most likely explanation.”
After the articles with the incorrect information circulated, a backlash against the parallel universe idea followed.
“Neil Turok and Luis Anchordoqui, these are serious physicists,” Gorham said. “I would never pooh-pooh anything they hypothesize, and science should not work that way. We look at hypotheses, we see if they’re testable and we try to make those tests.”
The future of neutrino astronomy
The next generations of both IceCube and ANITA may provide the most insight and information about neutrinos to date.
For ANITA, that’s PUEO and RNO. Vieregg, who was a graduate student on an early flight of ANITA, will be the principal investigator for both.
PUEO is the Payload for Ultra-High Energy Observations, similar in design to ANITA, and will also fly over Antarctica on a balloon. This instrument has benefited from the lessons learned during the ANITA experiment. The technology developed for PUEO is 10 times more sensitive and will allow scientists to dig even deeper in the search for neutrinos, “hopefully to discover what the universe has to offer at the highest energies in terms of neutrinos,” Vieregg said.
Radio Neutrino Observatory is a ground-based experiment that will place radio antennae across the ice sheet in Greenland. This placement on the ground will allow the researchers to look for lower energy particles that don’t make as bright of a signal, which overlaps some with IceCube.
And IceCube itself will get an upgrade, which is called IceCube Gen 2. Progress is being made on the “ongoing upgrade of the detector and our preparations for the next-generation instrument that will be an order of magnitude larger,” Halzen said.
Together, PUEO, RNO and IceCube2 will act as a set of observatories that cover the range of neutrinos and their energy ranges.
“What we’ve learned from ANITA and IceCube gives us the tools to build the next generation and do it in a way that gets to the science fast,” Vieregg said.
As Gorham looks ahead to the future of studying neutrinos, it unfurls more like a marathon than a sprint — but one that’s well worth it.
“What we do has horizons which are a generation long, that’s the benefit to society we have to be looking at,” Gorham said.
“When Pierre Curie discovered X-rays from radium that he put in his desk in the 1890s, he had no idea that by a generation later, X-rays would become the most valuable medical tool maybe ever, and they still are. If physicists hadn’t been working on quantum mechanics in the ’20s and ‘30s, we would never have gotten transistors in the ‘50s and ‘60s. We wouldn’t have cellphones and computers today.
“These things take a generation to really unfold in terms of their benefits, and that’s certainly the case for neutrinos. It may be another generation before we understand them, and we can’t predict what the outcome may be, but it probably will be incredible.”
The beginning of neutrino astronomy is happening now, Vieregg said. As researchers learn about the sources of these neutrinos, they can put the larger picture together.
And we’re also living in a time when multi-messenger astronomy — a combination of observations and data across the electromagnetic spectrum — is helping to shed light on previously mysterious occurrences in the universe. These messengers include the detection of gravitational waves, or ripples in space time, as well as the neutrino tracing detection by IceCube in 2018.
“We’ll be reaping the scientific rewards in the next 10 to 15 years,” Vieregg said. “Neutrinos are interesting because you can match them with other observations, such as optical from regular telescopes, or gravitational wave observations. Put them together and we can learn even more about what’s out there in the universe.”